Thermal Engine Cycle Using
Ideal Gas
Introduction
The usual
gas thermal engine cycle involves two isothermal excursions along with two
adiabatic excursions the last of which returns the working gas to its original
pressure and volume as depicted on a plot of pressure versus volume. In this animation I have chosen to plot
internal energy and volume separately versus time. At the same time I show the motion of the gas
particles and piston while plotting these parameters. I think this removes some of the mystery of
how isothermal and adiabatic excursions are achieved.
Figures:
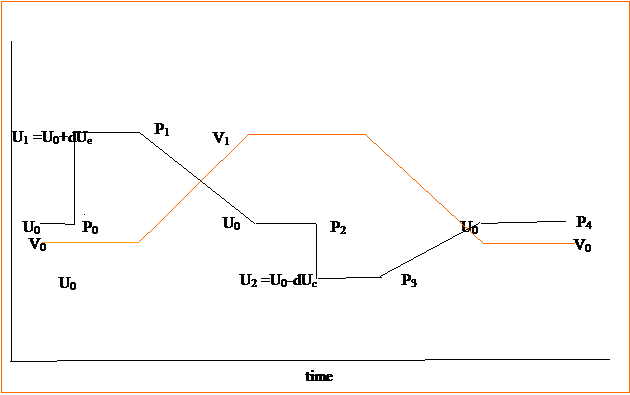
Figure 1: Internal energy, U and Volume, V as a
function of time over a complete cycle.
The cycle design adds heat at stage, then expansion at stage 2 back to
the original internal energy U0 .
Then the internal energy is reduced by putting the gas in contact with a
cooler region. Finally the
gas is compressed back to its original internal energy, volume, and pressure to
complete the cycle. Pressure is labeled
P0, P1, P2, P3 and P4 at
the various stages of the cycle.
Subscript e stands for expansion and c stands for contraction.

Figure 2:Animation experiment showing container and
piston and gas particles along with plots of internal energy, U (black),
pressure, P (blue), integral of Pdh (red) where h is piston height, calculated
internal energy (green), UT, and piston height (orange).
Calculation of Energy per Cycle
The ideal gas law is:
|
|
(1.1)
|
where c is a constant that depends on the ratio of heat
capacity at constant pressure to that at constant volume. For simple hard spheres and a 2 dimensional
gas like we are considering here c=1 while for a 3 dimensional gas c=2/3. The actual value of c will not be important
in the following calculations since these use only ratios of the quantities of
interest.
From equation (1.1)
we can write the pressures, P, at the various stages of the cycle as:
|
|
(1.2)
|
However, since in stage 4, both the volume and the internal
energy are the same as in stage 0, we must conclude:
|
|
(1.3)
|
|
|
(1.4)
|
We can solve for dUc in terms of U0
and dUe
|
|
(1.5)
|
Since dUe is greater than dUc we
deduce, from conservation of energy, that we can obtain macroscopic work
|
|
(1.6)
|
from the piston. The
amount of work can be obtained by solving line 2 of equation (1.5)
for dUe-dUc
|
|
(1.7)
|
The efficiency, η,
of the cycle is the work obtained divided by the amount of heat input
during the first part of the cycle:
|
|
(1.8)
|
Since U is always proportional to temperature we can rewrite
equation (1.8)
as:
|
|
(1.9)
|
Where TH is the highest temperature and T0
is the base temperature. Equation (1.9)
is recognizable as the expected relation between efficiency and
temperatures. Note, however, that this
treatment never brought up the mystical concept of entropy.
Calculation of Internal Energy during Expansion or Contraction
Both the internal energy and the pressure are changing
during volume change. Using equation (1.1)
we can write
|
|
(1.10)
|
|
|
(1.11)
|
where V0 is the starting volume and V is the
volume at any part of the volume change phase.
The red plot shows that the internal energy change is proportional to
|
|
(1.12)
|