Magnetic Forces from Special
Relativity
Introduction
It is possible to transform the speeds of both the fixed
positive charges in the wire as well as the moving negative charges in the wire
to a frame where the test particle is stationary. Then the magnetic forces on the test particle
disappear and we find that we are left with a net electric charge on the wire
segments that are parallel to the direction of the test particle motion and the
direction of the current on that wire segment.
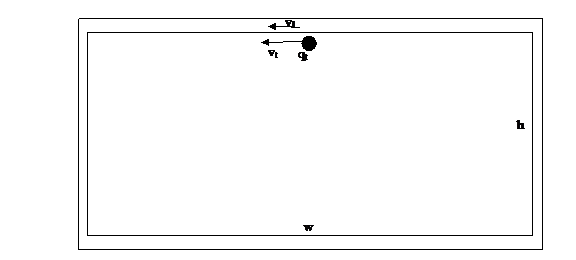
Figure 1: Current loop and test charge as viewed in
laboratory frame. The velocity of the
test charge is vt so its beta is vt/c. The velocity of the negative charge current
is vI.
Velocity Transformations
Looking at Figure 1 we see that prior to transformation:
|
|
(1.1)
|
After the transformation to set the test particle speed, vt,
to zero we have:
|
|
(1.2)
|
Let us define some value of
the various Lorentz factors, γ.
|
|
(1.3)
|
|
|
(1.4)
|
To compute the charge density for these betas we
must obtain their Lorentz boost .
|
|
(1.5)
|
After a bit of algebra we obtain the results:
|
|
(1.6)
|
Using definitions of the gammas of t and I equation (1.6)
becomes:
|
|
(1.7)
|
and then the final expression for the gamma of the charge
density becomes:
|
|
(1.8)
|
The positive charge spacing is contracted by the factor and the negative charge spacing is contracted
by the factor . Prior to transformation let the positive
charge per unit length be and the negative charge per unit length be .
You should recall that the negative charge was moving prior to transformation, and therefore
charge spacings were contracted by prior to the transformation so that the factor
must be multiplied by to get the effective negative charge density.
|
|
(1.9)
|
Then the new net charges per unit length
will be
|
|
(1.10)
|
Please note in equation (1.10)
that the sign of in the top side of the loop was opposite from that
in the bottom side of the loop. Looking
at equation (1.8),
that fact also results in quite different charge densities in the top and
bottom sides of the loop.
When the law for transformation of the force from the charge's
moving frame to the laboratory frame
|
|
(1.11)
|
is taken into account, the factor in equation (1.10)
is factored out and the new electric force is the same as the magnetic force
before transformation.
Charge Density in a Current Loop with a Finite number of Charges
Assume that
we have linear charge distributions in all 4 legs of a current carrying loop
and that we've transformed the charge velocities to make the test charge near
the top leg stationary.
To be definite, let's assume that the total number of
negative charges in the loop is Nc and, since the loop wire has no net
charge, the number of positive charges is also Nc. Since total charge number is a scalar, the
transformation discussed above cannot change this. The height of the loop remains the same
during the test particle transformation but its width is contracted by the
factor 1/γt. We will first compute the number of positive
charges in the loop sides and the top and bottom of the loop. We have the equation:
|
|
(1.12)
|
Charge Speed in a Current Loop
It is obvious that the rate or charge flow has to be
constant around the loop including at the corners where there are
discontinuities in the charge per unit length. That would dictate that the following
equation be valid
|
|
(1.13)
|
where n corresponds to the loop side number counted in the
direction of charge flow.
Recall from Figure 1 that both vt and vI
in the top of the loop are both shown going to the left which is the -x
direction. Therefore, before
transformation, we write:
|
|
(1.14)
|
As observed in the frame of the stationary test particle these
speeds become:
|
|
(1.15)
|
The transformed speed of all positive charges is vt as given in equation (1.15)
. Relative to the stationary test
particle, the speed of the negative charges in the left side of the loop is and that in the right side of the loop is . The transformed speeds of the negative
charges in the top side and bottom side of the loop are given by equation (1.15).