Paraphrase of Basics of Space
Flight
Introduction
The
link Basics of
Space Flight gives a thorough set of equations in terms of the burnout
speed, v1, the range, r1, from the center of a
large mass, GM, and the flight angle γ1 of the vector velocity with respect to the vector
range. From these we need to find the
value of the initial true anomaly angle ν1, the ellipse semi-major axis a, the ellipse
eccentricity e, and the ellipse
semi-minor axis b.
Figures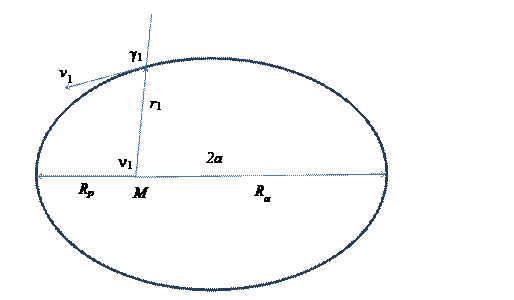
Equations
There are
two conservation laws that pertain to this problem: Energy and Angular
Momentum.
The energy law is
|
|
(1.1)
|
And the angular momentum law is:
|
|
(1.2)
|
At both the periapsis, Rp,
and the apapsis, Ra, ,
so equation (1.2) becomes:
|
|
(1.3)
|
Combining equations (1.3) and (1.1) we obtain the following
equations for the speeds at periapsis and apapsis
|
|
(1.4)
|
We can also use these expressions
to get Rp and Ra
|
|
(1.5)
|
Again using equation (1.2) for vp and Rp
we can write
|
|
(1.6)
|
And again using equation (1.1) we can write:
|
|
(1.7)
|
Which can be solved for Rp
by multiplying first by which results is the equation
|
|
(1.8)
|
Which results in
|
|
(1.9)
|
By definition of an ellipse with semi-major axis a and eccentricity e we have
|
|
(1.10)
|
These equations can be solved for
e by eliminating a
|
|
(1.11)
|
And we already have expressions for Rp and Ra in equation (1.9).
Also from equations (1.10) we have a result for the
semi-major axis
|
|
(1.12)
|
Which means that a
is independent of initial flight angle γ1.
A much more convenient expression for e is
|
|
(1.13)
|
We also need the “true anomaly” angle from
the periapsis point to the initial position vector end, r1, (see
Figure) and this is obtained from the equation
|
|
(1.14)
|
Another easier expression for a is
|
|
(1.15)
|
Also, since we have equation (1.13) for e, and equation (1.15) for a, we can obtain Rp
and Ra from equations(1.10) without the need for the
quadratic equation solution (1.9).
Parameters e and a specify the shape
of the ellipse while Rp
defines the distance of closest approach to the large mass, GM, and expresses the starting angle.
Note, for any ellipse, that the
semi-minor axis, b, is
|
|
(1.16)
|
We need one more expression: the
rate of increase of the true anomaly angle .
Three equations are involved
here. First one is called the eccentric
anomaly,E, and is
|
|
(1.17)
|
The second one is for the mean anomaly
angle, M
|
|
(1.18)
|
The third equation relates the change of M to the change of time:
|
|
(1.19)
|
So we solve equation (1.17) for E in terms of , then M
is related to E and the progression
of M with respect to time is
|
|
(1.20)
|
And using the chain rule we can write
|
|
(1.21)
|
And then use the chain rule on equation (1.17) to obtain and expression
for in terms of .
Referring to mean and eccentric anomaly as we did above is
more complicated than necessary. Instead
we refer to Kepler
Laws of Planetary Motion from which we obtain the following equations for
the period of the elliptical motion, T, distance from the large mass, r, and the rate of angular motion
|
|
(1.22)
|
|
|
(1.23)
|
|
|
(1.24)
|
Combining equations (1.16), (1.22), and (1.24) in equation (1.23) we get the following
expression for the rate of change
|
|
(1.25)
|
To obtain the angle and radius
r after a time t, we integrate
equation (1.25) with respect to
time. This (x,y) location of the smaller
mass, with respect to the center of the larger mass, is then
|
|
(1.26)
|